Microwave radiometry: a new non-invasive method for the detection of vulnerable plaque
Introduction
Atherosclerosis is a major cause of premature morbidity worldwide, while carotid artery atherosclerosis is the most important cause of ischemic stroke (1). Although in a large proportion of previously asymptomatic individuals, sudden cardiac death or ischemic stroke occurs as the first manifestation of atherosclerosis (carotid or coronary), most of the atherosclerotic plaques remain harmless for a long period of time. In order to identify in vivo the high-risk plaque we need to recognize the specific morphological and functional characteristics as recent studies have shown that even low-grade stenosis may lead to the development of acute events (2-4).
The morphological characteristics of the atheromatic plaques have been identified in vivo studies, and include: (I) a large lipid core (≥40% plaque volume) composed of free cholesterol crystals, cholesterol esters, and oxidized lipids impregnated with tissue factor (II) a thin fibrous cap depleted of smooth muscle cells and collagen (III) an outward (positive) remodeling (IV) inflammatory cell infiltration of fibrous cap and adventitia (mostly monocyte-macrophages, activated T cells and mast cells), and (V) increased neovascularization. The terms vulnerable, unstable or ‘high-risk’ are now widely used to describe plaques that exhibit such features (5). Currently, there is no ideal method for the identification of all these morphological characteristics (6). Last but not least, there are recent studies proving the role of a new morphologic characteristic, “intima media thickness variability” (IMVT) which found to be strongly correlated with cerebrovascular events (7).
However, the problem is greater in the identification of functional characteristics of the unstable plaque. The inflammatory activation of the atherosclerotic plaque is well defined and is also well known that the infiltration of the plaque by inflammatory cells, the lack of oxygen in ischemic areas and neovessel formation which increases blood flow contribute to increased heat production (8,9).
Based on these criteria, intravascular thermography (IVT) is an invasive method, which provides additional diagnostic and prognostic information in the identification of the high-risk atheromatic plaques in patients with coronary artery disease (10-27). However, the invasive character of the identification of the plaque of IVT, excludes this method from primary prevention.
Therefore the validation of a new non-invasive method that can identify the inflammatory activation in atheromatic plaques with similar accuracy as IVT, could be an alternative, simple method for the detection of the functional characteristics of the vulnerable atheromatic plaques. Microwave radiometry (MR) is a new method, based on the ability to detect noninvasively, with high accuracy, some of the functional characteristics of the plaque such as relative changes of temperature in human tissues (28-30).
Thermal heterogeneity of atherosclerotic plaques-basic principles of MR
Cascells et al. reported that there is increased temperature heterogeneity in ex vivo atherosclerotic specimen of human carotid arteries. Moreover, temperature heterogeneity was also confirmed by the use of an infrared camera in vivo and the most important was that the measured temperature was directly correlated with inflammatory cell density (31). These were the first attempts showing that heat production is strongly correlated with atheromatic plaques. There were also other preliminary clinical studies confirming the above (32,33). Although inflammation leads to increased heat release from the atherosclerotic plaque, higher temperature differences have been observed in ex-vivo compared to in-vivo temperature measurements (34). Measurements with IVT in abdominal aortas (35) showed that blood flow may exert a “cooling effect” on measured temperature which is also affected by cap thickness, source geometry and maximal flow velocity (36). Thus, there are several technical shortcomings of the available intravascular catheters and the evaluation of the diagnostic and prognostic implications of IVT is infeasible with the current technology.
Microwave radiometry (MR) is a new non invasive method that converts electromagnetic radiation from internal tissue at microwave frequencies and provides accurate measurement of the temperature of the patient’s internal tissue. MR measurements, performed with the RTM-01-RES microwave computer based system, are based on the principle that the intensity of the radiation is proportional to the temperature of tissue (37-43).
The system of MR possesses an antenna with 2 sensors: a microwave and an infrared. The microwave sensor is 3.9 cm in diameter and detects microwave radiation at 2 to 5 GHz, which corresponds to 7 cm in depth, with accuracy for temperature measurements of 0.20 °C. The sensor of MR filters all possible microwaves or radiofrequency waves that may be present in the room vicinity and may cause interference with the sensor (Figures 1,2). The “volume under investigation” has a rectangular area of 3 cm in width, 2 cm in length, and 3 to 7 cm in depth depending on the dielectric properties of the underlying tissues, the wavelength, and the water content of the tissue (40). The second sensor is used for infrared measurements from the skin, for calibrating the microwave sensor readings. The technical characteristics of MR are presented briefly in Table 1.
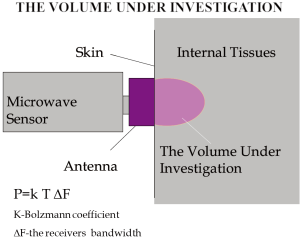
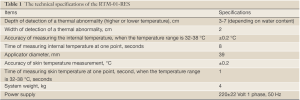
Full table
Microwave radiometry has already been applied in oncology for the detection of breast and thyroid cancers, and for monitoring of the treatment of benign tumors (28-30,44-48). Microwave Radiometry presents some advantages in the detection of breast cancer. First of all it is non-hazardous both to the patients and to the personnel taking the thermograms. Moreover, it is a non-invasive method and it detects thermal changes that precede to the anatomical changes detected by ultrasound, mammography and others. This early detection highlighted MR as a very promising method for breast cancer at an early stage. Last but not least, it presents two more advantages. Firstly, so fast growing tumors are “hotter” and they are more contrast in thermograms as the specific heat generation in the tumor is proportional to grow rate of the tumor. This allows MR to be the unique method to detect fast growing tumors and in conjunction with other traditional methods allows selecting patients with fast growing tumors (49).
History of microwave radiometry and basic principles
Non-invasive measurement of internal organ temperatures began one hundred years later due to the development of night vision equipment during the Second World War. By this method skin temperature was measured. The skin temperature partially reflects internal organ temperature, due to heat transfer. Twenty years later [1972] the first investigation of measuring internal tissue temperature were held. The measurement was based on receiving natural electromagnetic radiation at microwave frequencies. The first work that recognized microwave radiometry as a method for detecting breast cancer was published in 1977. However to this present day the method has not been used widely in medical practice.
Heat transfer occurs by tissue conduction as well as blood convection. However, from the physiologic viewpoint, it seems to be more appropriate to distinguish between the two following processes (I) “effective” conduction, including conduction in the physical sense (Fourier’s law) and convection by the capillary vessels assumed to be distributed isotropically (II) convection through the relatively large vessels, the veins essentially, according to Newton’s law. It is noteworthy that maximal capacity of heat transfer by convection through large vessels is much higher than by tissue conduction and capillary convection, up to 100 times, approximately. In some parts of a human body the temperature is constant due to homeostasis. For example, the central nervous system, thorax organs, the abdominal area, all have a constant temperature. When the room temperature is 20-25 °C the skin temperature lowers to 32-33 °C so there is a temperature gradient between the skin temperature and the internal temperature. According to the law of physics, any object above zero degrees Celsius emits radiation at all frequencies and, in particular, in the microwave region, that is used in microwave radiometry. This feature of heated objects is used for measuring averaged internal tissue temperature and detecting thermal abnormalities (higher or lower temperature of internal tissue). The noise power received by the antenna contacted an evenly heated absorbing object is:
Therefore the power noise received by the antenna is proportional to the tissue temperature. When the object temperature is 309 °K, i.e., 36 °C the noise power received by the antenna is 3×10-13 Watt. This value corresponds with the noise generated by the antenna. Special methods are applied for receiving and processing signals. In 1997 RES, Ltd. developed the RTM01RES computer based microwave radiometer which is safe, simple in operation and does not require a calibration procedure. The system consists of the following items: (I) Internal Temperature Sensor with the antenna (ITS) (II) Skin Temperature Sensor (STS) (III) Data Processing Unit (DPU). The system includes a personal computer and a printer. The device is connected to a PC through a serial port. The results of RTM-diagnosis are shown on the monitor of the computer or printed as a thermogram and temperature field on the projection of the investigated organ. When the temperature is measured, the antenna’s position on the patient’s skin is in accordance with the computer diagram of the examined organ. The antenna receives microwave radiation from the examined organ as noise at microwave frequencies and the signal is amplified in ITS. The signal amplified in ITS is transmitted to the DPU (data processing unit), where it is processed. The voltage from the skin temperature sensor (STS) is transmitted to the DPU too. The skin temperature sensor is non-contact infrared frequencies receiver. The buttons located on the front cover of the data processing unit switches the modes. The internal and skin temperature values are displayed on the 3-digit temperature indicator as degrees Celsius with an accuracy of 0.1. The Data processing unit produces a series of digital signals for interfacing with the PC. In some works discussing microwave radiometry temperature data is displayed as a diagram when the names of the measured points, go along the horizontal axis and the internal temperature values are along the vertical axis. This method allows analysis of temperature differential between corresponding points on the left and right breasts. However it is difficult to analyze the temperature at various locations on one entire breast by this method. Therefore the temperature data are also displayed as a temperature field, that is used in infrared thermography in the temperature field, cool areas of the breast are displayed by “cold” colours (i.e., blue) and hot ones are reflected by “warm” colours (red and orange). Internal temperature fields show temperature abnormalities, in particular, corresponding to the location of a cancer.
First application of MR in an experimental model
The first attempt to validate the measurements performed with the MR device with the measurements performed with the gold standard for assessment of thermal heterogeneity which is the IVT, was in an experimental model of atherosclerosis. Twenty four New Zealand White rabbits were used in this study. Twelve rabbits were randomly assigned to a normal diet, whereas 12 others were fed a cholesterol-rich diet (0.3% cholesterol and 4.7% coconut oil) for 6 months. At 6 months, all rabbits were prepared for intravascular angiography, IVT and MR of the abdominal aorta. The Institutional Laboratory Care and Use Committee approved the protocol which was conducted in accordance with regulatory guidelines for the care of laboratory animals. The procedure of the protocol was briefly the following: after the introduction of a 5Fr introducer sheath though the right carotid artery, angiography of the abdominal aorta was performed to ensure the presence of atherosclerotic plaques. Before temperature measurements the abdominal aortas were divided by optical coherence tomography (OCT) in 5 consequent segments with equal length (2 cm). External radiopaque markers were placed for the separation of the 5 segments. Thereafter, temperature measurements of the abdominal aortas were performed (I) invasively with IVT, and (II) noninvasively with MR (RTM-01-RES system, IMRI, Bolton University, Bolton, UK). Thermography was performed in 60 segments in each group, and we obtained 48 temperature differences in hypercholesterolemic rabbit aortas and 48 in the control rabbit aortas whereas a histological and immunochemistry analysis followed. There were no complications during the procedures. The main findings of this study were that microwave radiometry can measure non-invasively temperature differences in hypercholesterolemic rabbit aortas and the temperature measurements obtained by MR are correlated with intravascular measurements. Moreover, temperature differences of hypercholesterolemic segments assessed by both methods were correlated with plaque thickness and inflammatory infiltrates assessed by histology (35).
This study demonstrated that MR can provide temperature measurements of the arterial wall non-invasively. The current technological characteristics limit the use of MR in arteries with a depth <7 cm from the skin. Thus, the first in vivo application of MR in humans was performed in carotid arteries for the detection of inflamed plaques. The clinical implications of MR in the diagnosis and prognosis in carotid artery disease seemed wide.
First in vivo application of MR
After the validation of the method in an experimental model, the first application of MR in human carotids was proceeded in order to show the detection of thermal heterogeneity. Fortyfour consecutive patients with significant carotid artery stenosis were included in the study. Ten patients who met the exclusion criteria were excluded. At last, thirty-four patients undergoing carotid endarterectomy underwent screening of carotid atherosclerosis by ultrasound and MR. There was also a control group by healthy volunteers. During ultrasound study, plaque texture, plaque surface, and plaque echogenicity were analyzed. To ensure that matching cross sections were compared between ultrasound and MR, measurements were performed at each segment starting from the proximal common carotid artery and moving distally, based on skin markers located under the guidance of ultrasound. Moreover, the sensor of the antenna measured as already mentioned the ‘volume under investigation’ as a rectangular area of 3 cm in width and 2 cm in length, and 3-7 cm in depth depending on the water content of the body. Thermal heterogeneity (ΔT) was assigned as maximal temperature along the carotid artery minus minimum. Association of thermographic with ultrasound and histological findings was performed. For measurements of the temperature in each segment by MR, both intraobserver (0.06±0.08 °C; range, 0 to 0.20 °C) and interobserver (0.08±0.11 °C; range, 0 to 0.30 °C) differences were lower than the accuracy of the microwave sensor (r=0.94 for intraobserver and r=0.89 for interobserver measurements). ΔΤ was higher in atherosclerotic carotid arteries. Fatty plaques had higher ΔΤ compared to mixed and calcified. Plaques with ulcerated surface had higher ΔΤ compared to plaques with irregular and regular one. Heterogeneous plaques had higher ΔΤ compared to homogenous. Specimens with intense expression of CD3 had higher ΔΤ compared to specimens with low. Specimens with intense expression of CD68 had higher ΔΤ compared to specimens with low expression. Specimens with intense expression of VEGF had higher ΔΤ compared to specimens with low.
The main results of this study were that: (I) MR can measure non-invasively thermal heterogeneity of the carotid atheromatic plaques in vivo, (II) the in vivo temperature measurements by MR correlated well with the ultrasound atherosclerotic plaque characteristics, the histological and immunohistochemical findings. All these suggested that MR may ultimately allow the noninvasive detection of plaque inflammation in vivo. Microwave radiometry, may be potentially used to identify patients with a moderate degree of carotid artery stenosis who might benefit from closer monitoring, more aggressive medical therapy, or early intervention (50). The fact that in 21% of the plaques without any ultrasound criterion for vulnerability, high temperature was found in accordance with the histology justifies more prospective clinical studies for the investigation of the prognostic value of temperature difference in carotid artery disease.
Other clinical studies with in vivo measurement of plaque neovascularization and thermal heterogeneity
Carotid artery stenosis is a poor predictor for future stroke and there are also other characteristics of the atheromatous plaques that cannot be detected by ultrasound. A potential positive feedback loop between plaque neovascularization and inflammatory activation has emerged (51). To this direction, several non-invasive methods have been developed for the identification of neovascularization and plaque inflammation. A new-non invasive imaging modality (CEUS), has emerged to provide direct visualization of plaque neovascularization, using contrast microspheres as intravascular tracers (52-54). Moreover, MR detects plaque inflammation by measuring atheromatous plaque temperature (38). Recently, we demonstrated in carotid atheromatous plaques of patients with coronary artery disease that plaque neoangiogenesis as this is assessed by CEUS, correlated with plaque temperature as measured by MR, and ultrasound plaque characteristics. Briefly, in this protocol, consecutive patients undergoing coronary angiography for chest pain evaluation suggestive of ischemic heart disease were prospectively enrolled in the study but forty-eight patients (64.8%) finally met the inclusion criteria and 86 carotid arteries were analyzed. All participants underwent standard carotid ultrasound examination followed by CEUS and MR measurement (16). In previous studies, it has already been proved that CEUS provides direct visualization of the adventitial vasa vasorum and intraplaque neovascularization (55,56) whereas MR provides accurate temperature measurements and is associated with histological inflammatory infiltration. We have already shown that plaque neoangiogenesis as assessed by CEUS, correlated with plaque temperature as measured by MR, and ultrasound plaque characteristics. The neoangiogenesis and inflammatory activity have already been proved to identify vulnerable plaque. The last clinical study demonstrated this correlation in intermediate lesions of patients without cerebrovascular events. Thus, a clinical study with serial CEUS and MR measurements is justified in patients with intermediate lesions, and the combination of these new non-invasive imaging modalities may prove a tool for primary and secondary prevention of cerebrovascular and/or coronary events. Furthermore, CEUS and MR are two modalities targeting different characteristics of vulnerable plaque: neovascularization and inflammation. Although, a good correlation was found between these two characteristics, there is no ‘gold standard’ method for the in vivo quantification of neovascularization and/or inflammation. More clinical studies need to be done.
Conclusions
Microwave radiometry can be safely applied for the non-invasive measurement of arterial wall temperature, reflecting the local inflammatory activation as already proved in both experimental and clinical studies. This new method seems to eliminate the limitations of current technology for the in vivo quantification of inflammation in atherosclerotic plaques whereas is also strongly correlated with other new non-invasive imaging modalities such as CEUS for the identification of vulnerable plaque.
Acknowledgements
Disclosure: The authors declare no conflict of interest.
References
- Roger VL, Go AS, Lloyd-Jones DM, et al. Heart disease and stroke statistics--2012 update: a report from the American Heart Association. Circulation 2012;125:e2-e220.
- Chaturvedi S, Bruno A, Feasby T, et al. Carotid endarterectomy--an evidence-based review: report of the Therapeutics and Technology Assessment Subcommittee of the American Academy of Neurology. Neurology 2005;65:794-801.
- Liapis CD, Bell PR, Mikhailidis D, et al. ESVS guidelines. Invasive treatment for carotid stenosis: indications, techniques. Eur J Vasc Endovasc Surg 2009;37:1-19.
- Hobson RW 2nd, Mackey WC, Ascher E, et al. Management of atherosclerotic carotid artery disease: clinical practice guidelines of the Society for Vascular Surgery. J Vasc Surg 2008;48:480-6.
- Naghavi M, Libby P, Falk E, et al. From vulnerable plaque to vulnerable patient: a call for new definitions and risk assessment strategies: Part II. Circulation 2003;108:1772-8.
- Tan KT, Lip GY. Imaging of the unstable plaque. Int J Cardiol 2008;127:157-65.
- Saba L, Mallarini G, Sanfilippo R, et al. Intima Media Thickness Variability (IMTV) and its association with cerebrovascular events: A novel marker of carotid atherosclerosis? Cardiovasc Diagn Ther 2012;2:10-8.
- Tenaglia AN, Peters KG, Sketch MH Jr, et al. Neovascularization in atherectomy specimens from patients with unstable angina: implications for pathogenesis of unstable angina. Am Heart J 1998;135:10-4.
- Madjid M, Willerson JT, Casscells SW. Intracoronary thermography for detection of high-risk vulnerable plaques. J Am Coll Cardiol 2006;47:C80-5.
- Toutouzas K, Synetos A, Stefanadi E, et al. Correlation between morphologic characteristics and local temperature differences in culprit lesions of patients with symptomatic coronary artery disease. J Am Coll Cardiol 2007;49:2264-71.
- Toutouzas K, Tsiamis E, Vavuranakis M, et al. Coronary artery plaque temperature: what do we measure? Eur Heart J 2004;25:993-4.
- Toutouzas K, Markou V, Drakopoulou M, et al. Increased heat generation from atherosclerotic plaques in patients with type 2 diabetes: an increased local inflammatory activation. Diabetes Care 2005;28:1656-61.
- Toutouzas K, Markou V, Drakopoulou M, et al. Patients with type two diabetes mellitus: increased local inflammatory activation in culprit atheromatous plaques. Hellenic J Cardiol 2005;46:283-8.
- Toutouzas K, Drakopoulou M, Stefanadi E, et al. Intracoronary thermography: does it help us in clinical decision making? J Interv Cardiol 2005;18:485-9.
- Toutouzas K, Drakopoulou M, Mitropoulos J, et al. Elevated plaque temperature in non-culprit de novo atheromatous lesions of patients with acute coronary syndromes. J Am Coll Cardiol 2006;47:301-6.
- Toutouzas K, Drakopoulou M, Aggeli C, et al. In vivo measurement of plaque neovascularisation and thermal heterogeneity in intermediate lesions of human carotid arteries. Heart 2012;98:1716-21.
- Stefanadis C, Tsiamis E, Vaina S, et al. Temperature of blood in the coronary sinus and right atrium in patients with and without coronary artery disease. Am J Cardiol 2004;93:207-10.
- Stefanadis C, Toutouzas K, Vavuranakis M, et al. New balloon-thermography catheter for in vivo temperature measurements in human coronary atherosclerotic plaques: a novel approach for thermography? Catheter Cardiovasc Interv 2003;58:344-50.
- Stefanadis C, Toutouzas K, Vaina S, et al. Thermography of the cardiovascular system. J Interv Cardiol 2002;15:461-6.
- Stefanadis C, Toutouzas K, Vavuranakis M, et al. Statin treatment is associated with reduced thermal heterogeneity in human atherosclerotic plaques. Eur Heart J 2002;23:1664-9.
- Stefanadis C, Toutouzas K, Tsiamis E, et al. Thermography of human arterial system by means of new thermography catheters. Catheter Cardiovasc Interv 2001;54:51-8.
- Stefanadis C, Toutouzas K, Tsiamis E, et al. The cooling effect of coronary blood flow on heart: a new approach. J Invasive Cardiol 2004;16:455-8.
- Stefanadis C, Toutouzas K, Tsiamis E, et al. Increased local temperature in human coronary atherosclerotic plaques: an independent predictor of clinical outcome in patients undergoing a percutaneous coronary intervention. J Am Coll Cardiol 2001;37:1277-83.
- Stefanadis C, Toutouzas K, Tsiamis E, et al. Identification and stabilization of vulnerable atherosclerotic plaques: the role of coronary thermography and external heat delivery. Indian Heart J 2001;53:104-9.
- Stefanadis C, Toutouzas K, Tsiamis E, et al. Thermal heterogeneity in stable human coronary atherosclerotic plaques is underestimated in vivo: the “cooling effect” of blood flow. J Am Coll Cardiol 2003;41:403-8.
- Stefanadis C, Diamantopoulos L, Vlachopoulos C, et al. Thermal heterogeneity within human atherosclerotic coronary arteries detected in vivo: A new method of detection by application of a special thermography catheter. Circulation 1999;99:1965-71.
- Stefanadis C, Diamantopoulos L, Dernellis J, et al. Heat production of atherosclerotic plaques and inflammation assessed by the acute phase proteins in acute coronary syndromes. J Mol Cell Cardiol 2000;32:43-52.
- Barrett AH, Myers PC, Sadowsky NL. Microwave thermography in the detection of breast cancer. AJR Am J Roentgenol 1980;134:365-8.
- Shaeffer J, El-Mahdi AM, Carr KL. Cancer detection studies using a 4.7 Gigahertz radiometer. Cancer Detect Prev 1981;4:571-8.
- Shaeffer J, El-Mahdi AM, Carr KL. Thermographic detection of human cancers by microwave radiometry. Prog Clin Biol Res 1982;107:509-21.
- Casscells W, Hathorn B, David M, et al. Thermal detection of cellular infiltrates in living atherosclerotic plaques: possible implications for plaque rupture and thrombosis. Lancet 1996;347:1447-51.
- Madjid M, Naghavi M, Malik BA, et al. Thermal detection of vulnerable plaque. Am J Cardiol 2002;90:36L-39L.
- Naghavi M, John R, Naguib S, et al. pH Heterogeneity of human and rabbit atherosclerotic plaques; a new insight into detection of vulnerable plaque. Atherosclerosis 2002;164:27-35.
- Verheye S, De Meyer GR, Krams R, et al. Intravascular thermography: Immediate functional and morphological vascular findings. Eur Heart J 2004;25:158-65.
- Toutouzas K, Grassos H, Synetos A, et al. A new non-invasive method for detection of local inflammation in atherosclerotic plaques: experimental application of microwave radiometry. Atherosclerosis 2011;215:82-9.
- Madjid M, Willerson JT, Casscells SW. Intracoronary thermography for detection of high-risk vulnerable plaques. J Am Coll Cardiol 2006;47:C80-5.
- Williams LE, Springer EB. Microwave radiation: environmental impact and medical application. Minn Med 1981;64:593-9.
- Mizushina S, Shimizu T, Sugiura T. Non-invasive thermometry with multi-frequency microwave radiometry. Front Med Biol Eng 1992;4:129-33.
- Röösli M, Rapp R, Braun-Fahrländer C. Radio and microwave frequency radiation and health--an analysis of the literature. Gesundheitswesen 2003;65:378-92.
- Barrett AH, Myers PC. Subcutaneous temperatures: a method of noninvasive sensing. Science 1975;190:669-71.
- Leroy Y, Bocquet B, Mamouni A. Non-invasive microwave radiometry thermometry. Physiol Meas 1998;19:127-48.
- N’Guyen DD, Mamouni A, Leroy Y, et al. Simultaneous microwave local heating and microwave thermography. Possible clinical applications. J Microw Power 1979;14:135-7.
- Barrett AH, Myers PC. Microwave thermography: a method of detecting subsurface thermal patterns. Bibl Radiol 1975;6:45-56.
- Myers PC, Barrett AH, Sadowsky NL. Microwave thermography of normal and cancerous breast tissue. Ann N Y Acad Sci 1980;335:443-55.
- Lee JW, Lee SM, Kim KS, et al. Experimental investigation of the mammary gland tumour phantom for multifrequency microwave radio-thermometers. Med Biol Eng Comput 2004;42:581-90.
- Davis SK, Van Veen BD, Hagness SC, et al. Breast tumor characterization based on ultrawideband microwave backscatter. IEEE Trans Biomed Eng 2008;55:237-46.
- Bardati F, Iudicello S. Modeling the visibility of breast malignancy by a microwave radiometer. IEEE Trans Biomed Eng 2008;55:214-21.
- Helmy A, Holdmann M, Rizkalla M. Application of thermography for non-invasive diagnosis of thyroid gland disease. IEEE Trans Biomed Eng 2008;55:1168-75.
- Madjid M, Willerson JT, Casscells SW. Intracoronary thermography for detection of high-risk vulnerable plaques. J Am Coll Cardiol 2006;47:C80-5.
- Toutouzas K, Grassos C, Drakopoulou M, et al. First in vivo application of microwave radiometry in human carotids: a new noninvasive method for detection of local inflammatory activation. J Am Coll Cardiol 2012;59:1645-53.
- Fleiner M, Kummer M, Mirlacher M, et al. Arterial neovascularization and inflammation in vulnerable patients: early and late signs of symptomatic atherosclerosis. Circulation 2004;110:2843-50.
- Hilty KC, Steinberg DH. Vulnerable plaque imaging-current techniques. J Cardiovasc Transl Res 2009;2:9-18.
- Giannoni MF, Vicenzini E, Citone M, et al. Contrast carotid ultrasound for the detection of unstable plaques with neoangiogenesis: a pilot study. Eur J Vasc Endovasc Surg 2009;37:722-7.
- Owen DR, Shalhoub J, Miller S, et al. Inflammation within carotid atherosclerotic plaque: assessment with late-phase contrast-enhanced US. Radiology 2010;255:638-44.
- Shah F, Balan P, Weinberg M, et al. Contrast-enhanced ultrasound imaging of atherosclerotic carotid plaque neovascularization: a new surrogate marker of atherosclerosis? Vasc Med 2007;12:291-7.
- Coli S, Magnoni M, Sangiorgi G, et al. Contrast-enhanced ultrasound imaging of intraplaque neovascularization in carotid arteries: correlation with histology and plaque echogenicity. J Am Coll Cardiol 2008;52:223-30.