Insight from imaging on plaque vulnerability: similarities and differences between coronary and carotid arteries—implications for systemic therapies
Introduction
Atherosclerotic disease is the underlying process of two of the most important causes of death worldwide: ischemic stroke (IS) and myocardial infarction (MI) (1). Atherosclerosis has a high prevalence in western countries with values up to 75% of men and 62% of women aged 65 years and older (1). Despite significant advances in treatment, current conventional screening and diagnostic methods are insufficient to identify the victims before the event occurs even if in the last years the recognition of the role of the vulnerable plaque has opened new windows of opportunity in the field of cardiovascular medicine.
The two vascular territories responsible for most of the cardiovascular events are the coronary arterial tree and extracranial carotid arteries. In both carotid and coronary arteries, the rupture or fissuration of the plaque is considered the trigger of the thrombotic (in coronary arteries) or embolic (in carotid arteries) sequences that determine the MI or IS (2). However, the percent reduction in luminal diameter of the artery is still considered a dominant predictor for the stratification of the severity of atherosclerotic process; this is because stenosis quantification has been the only parameter and endpoint that could be non-invasively detected with in vivo imaging techniques in the past decades in the trials that assessed diagnostic yield of imaging methods and the risk/benefits of the revascularization treatments.
Nevertheless, multiple ex-vivo studies demonstrated that there are significant differences in terms of risk of plaque rupture given the same degree of luminal stenosis and that these differences are related to the differences in plaque structure (2-5). In fact, by focusing the attention to some plaque features such as intra-plaque hemorrhage (IPH), it was found an association with a significantly increased risk of plaque rupture and distal embolization (6).
These features can nowadays be explored in vivo, thanks to the evolution of advanced diagnostic methods. The clinical implementation of advanced vascular imaging allowed also to explore newer concepts such as: (I) similarities and differences in atherosclerotic process in coronary and carotid arteries; (II) potential to monitor the process of progression/regression of atherosclerotic plaque features (i.e., calcification, volume, remodeling, lipid content,…) (7); (III) understand the effect of drug therapies and potential monitoring of the plaque in order to optimize the risk stratification and to identify optimal treatment.
Computed tomography (CT) and magnetic resonance (MR) are the main non-invasive tools for plaque analysis both in carotid and coronary arteries, whereas other methods such as optical coherence tomography (OCT), and intravascular ultrasound (IVUS) are the main invasive intravascular methods for the assessment of coronary atherosclerosis; conventional doppler-ultrasound (US) plays also a major role in the assessment carotid artery atherosclerotic disease. A minor, but very intriguing role, might be played by nuclear medicine techniques and in particular by positron emission tomography (PET), which is the tool that at this point in time can bring us the closest to actual molecular/metabolic imaging of carotid and eventually coronary atherosclerosis. In simple words, PET might soon be able to provide information about metabolic activity of atherosclerotic plaques through the application of highly specific probes able to indicate plaque activity/vulnerability; this particular and very demanding application might also be in the future a territory that CT and MR might explore with probes developed with the same strategy.
Three decades have passed since greyscale IVUS presentation by Paul Yock and nowadays second-generation intra-vascular imaging techniques such as IVUS, radio frequency tissue characterization, OCT, and near-infrared spectroscopy (NIRS) have moved beyond research settings and are currently used during daily clinical practice.
In this review, we discuss the similarities and differences between coronary and carotid artery vulnerable plaque from the imaging point of view and the potential implications for systemic therapies according to the emerging evidences of the literature.
Search strategy and selection criteria
The references for this paper were derived from databases maintained by the authors and supplemented by PubMed (National Center for Biotechnology Information, U.S. National Library of Medicine, Bethesda, Md, USA) searches and no time restriction for publication date was used The search terms “Plaque”, “Coronary”, “Imaging”, “CT”, “MR”, “US”, “IVUS”, “PET”, “OCT” and “Molecular Imaging” were used. Review articles and animal studies were included. To restrict the number of references cited, priorities were given to original observations, multi-centre studies, and randomised trials. Additionally, review articles and animal studies that included comprehensive bibliographies were cited when appropriate. There were no language restrictions. The final reference list was generated on the basis of relevance to the topics covered in this Review.
Imaging plaque feature of vulnerability
Several retrospective autopsy and cross-sectional clinical studies have suggested that acute coronary syndromes as well as cerebrovascular events are caused by specific plaque features of vulnerability (8-10). There have been great advances in our knowledge of the cellular biology regarding the formation and progression of atherosclerotic plaques; currently, the identification of those features, associated with an increased risk of plaque rupture, is the target of several imaging research studies with multiple imaging techniques.
Atherosclerotic disease is a complex and heterogeneous process requiring decades to show evidence as plaque. The first radiologically detectable phases are characterized by the increased thickness of the arterial wall due to accumulation of foam cell histological changes in the intima layer. After years, a disproportionate increase of the wall determined the growth of a plaque that can represent a trigger for the development of embolic event. The atherosclerotic plaque can show several types of components (Intra-plaque hemorrhage; lipid core; necrotic areas, clusters of calcium) with concomitant presence of inflammation and/or neovascularization that represent the marker of the plaque’s activity. All these features can be present together or one of these can be disproportionately represented by explaining the different “subtypes” of plaque and the sub-sequent different profile of risk.
The unstable atherosclerotic plaque is of great importance because it is the primary culprit in acute coronary syndromes and ischemic stroke; advanced imaging modalities offer images with high-spatial resolution of the plaque thereby allowing to characterize its tissue composition. Several imaging studies have been performed in carotid arteries in the first place, because of the larger vessel size, superficial location, and lack of motion, as compared to the coronary arteries, and it has been demonstrated that specific plaque features are associated with an increased risk of plaque rupture: the most important are intra-plaque hemorrhage (IPH) the thin/ruptured fibrous cap (FC), presence and size of the lipid-rich-necrotic-core (LRNC), intra-plaque neovascularization (IPN) and plaque inflammation.
In carotid arteries, US, CT and MR techniques provide very good results whereas in coronary imaging the plaque analysis is still challenging and follows a more semiquantitative approach (Figure 1). In coronary arteries, OCT and IVUS are considered clinical standard for the assessment of in vivo plaque features. The combination of better resolution and flushing (typically with intravascular iodinated iso-osmolar contrast material) in OCT provides a clear interface between lumen and plaque surface. Conversely, one of the most useful features of IVUS is visibility of the full thickness of the vessel wall till the adventitial layer.
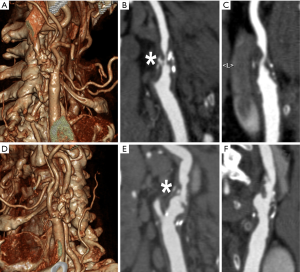
Degree of stenosis
Degree of stenosis was considered for both coronary and carotid artery plaques the key parameter to quantify the atherosclerotic severity of the disease. This is due to the fact that for decades the only modality able to directly evaluate arteries “in vivo” was conventional angiography (CA); however, CA could offer only a luminographic view of the vessels. Luminal stenosis was the only indirect parameter indicating the presence of atherosclerosis. This concept is nowadays completely changed because it is demonstrated that carotid artery wall develops a significant positive remodeling, while determining a mild degree of stenosis, that can subtend a focal eccentric large plaque volume (i.e., also referred as plaque burden). However, for carotid artery, percent reduction in luminal diameter is considered the key parameter for stratifying subsequent stroke risk and for selecting the optimal therapeutic approach (11); this in spite of the fact that strong evidence demonstrates that the degree of stenosis is a weak parameter to stratify the risk of acute events. Similarly, in coronary arteries, most lesions responsible for MI were angiographically mild at baseline [mean (±SD) diameter stenosis, 32.3%±20.6%] (12). The consolidation of this knowledge have led to separate the clinical situation of stable and unstable coronary artery disease. In the first case there is one or more coronary artery stenosis that may cause inducible ischemia (clinically evident with angina or angina equivalents such as dyspnea under exertion) under stress conditions but not quite likely to determine an actual acute myocardial infarction. In the second case, a non-significantly obstructive plaque, develops an intimal erosion/fissuration/rupture which leads to an acute intravascular thrombosis; this acute partial or complete blockage of the coronary flow determines at first acute rest ischemia of the ventricular wall which over time becomes myocardial necrosis.
Luminal morphology
The luminal morphology is considered a risk feature for both MI and IS (13). It is possible to define the surface of the plaque according to three classes: (I) regular; (II) irregular and (III) ulcerated. The luminal surface of the plaque corresponds to the intimal layer the artery wall. Several studies have demonstrated that the presence of irregular luminal surface in coronary arteries and in carotid arteries is associated with an increased risk of plaque’s rupture (14,15).
Therefore, the detection of intimal plaque irregularities is a parameter of vulnerability (i.e., increased risk complications such as rupture). In coronary arteries it is possible to identify the intimal irregularities using conventional coronary angiography (15), even though a significantly better performance can be obtained with more advanced intravascular techniques such as the OCT and IVUS due to higher spatial resolution, in particular in the intimal-medial layers (16,17). In carotid arteries the optimal imaging technique for luminal surface evaluation is CT because of the higher spatial resolution compared to MR (18,19). Also US offers information regarding the carotid plaque surface but the presence of calcium components may significantly limit the sensitivity of this techniques (20).
The ulceration of the plaque is accepted for carotid artery, as a prominent risk of vulnerability from the NASCET Trial. The impact of ulceration in carotid artery plaque determining mild stenosis is incorporated in the recently published ESC guidelines (21) whereas the management of non-obstructive ulcerated plaques in coronary arteries remains unclear, and clinical practice guidelines are not available.
Because of the size of the plaque of carotid arteries, the occurrence of carotid plaque ulceration is nowadays easily detectable with CT and MR imaging (22,23). In coronary arteries this task is more difficult, especially with non-invasive methods (i.e., CT); however, post mortem studies have robustly demonstrated this association (24,25).
IVUS and OCT can demonstrate with very good sensitivity and specificity this condition, even though for larger ulcerations also conventional coronary angiography can demonstrate this condition (26).
Schoenhagen et al. showed with IVUS that the prevalence of intimal ulceration in coronary arteries was significantly higher in patients with acute MI as compared to patients with stable/subacute group (19% versus 4%; P=0.014); in addition, there were no significant differences concerning other morphological lesion characteristics (8).
The luminal morphology represents the surface appearance in the inner lumen of the plaque and represents in both coronary and carotid arteries an important parameter of vulnerability (27). In particular, the more severe the intimal irregularities, the higher the risk of acute rupture and hence of acute ischemic events.
Plaque composition
In coronary artery disease (CAD), although large plaques are more likely to rupture than small plaques, the greatest risk of MI derives from the rupture of small plaques. This apparent paradox could be explained as follows: small plaques are far more common than large plaques and obstructive plaques (28); when there is already a significant reduction of flow due to a large plaque, coronary arterial circulation develops collaterals thereby reducing the impact of an acute blockage of coronary flow at the same site. In addition, some feature of the composition of smaller plaques contribute to their structural instability and thus to their rupture. The plaque composition represents the main target of the advanced imaging techniques because it allows to non-invasively assess plaque inner structure, ultimately allowing to detect and better distinguish high risk features from low risk features.
Plaque composition: calcium
Atherosclerotic calcification has intrigued researchers for more than a century and despite extensive research in this area, the mechanistic understanding of atherosclerotic calcification in humans remains limited (29). Heavy coronary calcifications can actually be detected even on a conventional chest X-ray. Because of the strong tissue attenuation calcium was the first tissue explored in coronary artery plaques also when the CT technology was not used for the analysis of coronary arteries due to excessive motion artefact (20,30). In fact it was electron beam computed tomography (EBCT), and not CT, the first imaging method able to provide adequate temporal resolution for a proper assessment of coronary artery calcifications. In the seminal paper by Agatston et al. (20) of 1990, it was found that the absence of detectable coronary artery calcium [i.e., a Coronary Artery Calcium Score (CACS) with Agatston method =0] had a negative predictive value of 98%. This concept that is today referred to as the “Power of Zero” should be amended by an important observation; the absence of detectable coronary calcifications (CACS =0) does not warrant the absence of non-calcified plaques. With the evolution of fast spiral CT which developed into multislice CT technology, the detection and quantification of CACS became much easier; currently CACS detected by cardiac CT (CCT) is considered representative of the burden of coronary atherosclerosis and it is effective for advanced and individualized stratification of cardiovascular risk in asymptomatic patients (31) beyond conventional global cardiovascular risk assessment algorithms (32). It is quite well demonstrated that subject with CACS value >100 have a significantly increased risk of MI compared to the CACS 0 Class and CACS 1–100 Class. However, not all the authors are concordant to consider coronary artery calcification as a predictor of plaque instability or vulnerability and they might be right in this (33). In fact, CACS location does not correlate with the site of future plaque rupture and events and also it does not correlate with the location of significantly obstructive coronary artery stenosis (i.e., lumen reduction >50%). Actually, when coronary artery calcifications are not accompanied with significant non-calcified plaque burden (i.e., lipid and or fibrous atherosclerosis of coronary artery walls) it is believed that this represents a quite stable and relatively “protective” condition against coronary events, especially in older individuals. In fact, very high CACS values (>1,000) are considered somehow protective in this context.
Interestingly, in carotid arteries, several papers showed the “protective” effect of calcified plaques (34-36), also in subjects with severe degree of stenosis. A meta-analysis (37) showed a significant negative relationship between calcified plaque and ipsilateral ischemia (OR, 0.5; 95% CI, 0.4–0.7).
Possible explanations of this phenomenon are probably to be researched in biology and pathophysiology of atherosclerosis. Recently, a study demonstrated that in highly calcified carotid plaques, miRNAs may play a modulating role in calcified plaques and in plaque stability (38); another recent study identified two types of calcium salts in the atheromatous carotid plaques: hydroxyapatite and calcium oxalate. Hydroxyapatite calcification was associated with more unstable plaques while calcium oxalate calcifications were mainly detected in stable plaques (39). This evidence may suggest that calcium should not be considered as unique entity with only quantitative features, but also with qualitative features.
Plaque composition: IPH and LRNC
In both carotid and coronary arteries, the presence of LRNC and/or of IPH are considered indicators of plaque vulnerability and hence risk factors for plaque rupture. The lipid core results from the death of lipid-laden macrophages, or foam cells, and from the accumulation of lipids in the extracellular matrix. Erythrocyte membranes, which have a very high cholesterol content, may also contribute to the inflammatory response and to the accumulation of free cholesterol in coronary plaques (5). Lipid composition is believed to influence the stability of atherosclerotic plaques, given that the level of free cholesterol is significantly increased in disrupted lesions (40). Not the presence but the percentage of LRNC is related to the risk of rupture and those plaques whose lipid core exceeds 40% of the volume are especially vulnerable in coronary and carotid arteries (41). It has been showed that the thickness of lipid component is significant associated with the complicated AHA type VI plaque is (ROC AUC =0.89) (42).
The identification of LRNC is difficult and might be possible with very few imaging techniques; in particular, MR is considered a suitable imaging technique to explore carotid artery plaque composition because it can properly identify the tissue composition, given its multi-parametric capabilities which allows the differentiation between fat/lipid tissue and other tissues. However, standard 1.5 Tesla MR equipment is not routinely used to identify the presence of LRNC because of some technical limitations mainly related to motion artefacts. However, a recently published study demonstrated that using 3 Tesla MR equipment can more accurately detect LRNC (43).
CT, which is mostly a mono-parametric method, can be easily used for both coronary and carotid plaque assessment of areas of low attenuation (<60 HU) corresponding to lipid rich plaques (Figure 2). US (44,45) in carotid arteries and IVUS in coronary arteries show that hypo-echogenic plaques are associated with the presence of LRNC.
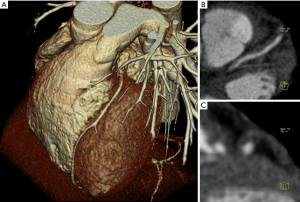
The association between IPH and progression of coronary lesions was suggested in 1938 by Wartman et al. (28) and interestingly it is hypothesized that IPH could represent the basis for the formation of the LRNC (46) (because of the substantial amount of lipid content derived from the erythrocyte membranes) and, at the same time, to represent a powerful plaque inflammatory trigger because erythrocyte membranes can promote the recruitment of macrophages under the fibrous cap. Because of these findings, currently, IPH represents a critical event in the induction of instability in these lesions.
Also for the carotid arteries, IPH is considered one of the identifying features of vulnerable plaque and 2 recently published meta-analyses found that carotid IPH is associated with increased risk for future primary and recurrent ischemic neurological events (47,48). US and CT have low sensitivity and specificity in the detection of IPH (49) although some studies have suggested that low HU value in CT (25 HU) (50,51) is associated with the presence of IPH. Some recently presented techniques, such as near-infrared autofluorescence seems to be promising for the in vivo monitoring of IPH (52).
Plaque composition: neovascularization and inflammation
A further parameter related to the plaque vulnerability is the “plaque activity” that identifies the level of inflammation and neovascularization of the plaque. In normal arteries microvessels (vasa vasorum) are detected in the only adventitia and outer media layers but when neovascularization occurs, a double modality it is possible: from the adventitia, and from the arterial lumen, into the intima of advanced plaques. These microvessels are characterized by immature endothelial lining with an increased probability of leakages and IPH formation with transition from a stable to an unstable/vulnerable plaque.
In coronary arteries, pathological study (5) found that advanced lesions often contain extensive areas of neovascularization and several researchers have found that IPH is associated with an increased density of microvessels (53,54) and similar results were also found for carotid arteries (55,56) by implying that the IPH significance is similar for carotid and coronary arteries. A surrogate for intra-plaque neovascularization is the so called “napkin-ring sign” which represent the layer of iodinated contrast material on the outer contour (i.e., adventitia) of the non-calcified component of atherosclerotic coronary artery plaque (PAL ref.). However, given the size of the coronary arteries and of the plaques it is still quite difficult to gain significant insight into this phenomenon with non invasive tools such as CT.
A difference in imaging approach is present because neovascularization can be adequately studied with CT, MR and CE-US (57-61) whereas the IPH of coronary arteries nowadays can be actually explored only with OCT or IVUS (62-64).
Recurrent events scenario
A further challenging question is the approach to patient that have suffered MI or IS. The estimated risk of stroke is 12% to 20% during the first 3 months after a transient ischemic attack (TIA) or minor stroke whereas in the acute coronary syndrome risk is 8–10% (65).
The Providing Regional Observations to Study Predictors of Events in the Coronary Tree (PROSPECT) trial (12), which studied coronary plaques using IVUS in survivors of acute coronary syndrome, found that plaque characteristics such as thin/rupture FC and a plaque burden >70%, were associated with 2.5- to 5-fold increased risk of repeated events.
Similarly, papers have shown that the risk of stroke during the first 90 days after a TIA or minor stroke ranges between 3.7% and 11.7% (65-67). In the CARE II trial (52) about one sixth of the symptomatic subjects have in the contra-lateral carotid artery features of vulnerability suggesting an increased risk of rupture and events.
Identification of specific features that significantly increase the recurrent risk of plaque rupture represent an important need for the therapeutic stratification with authors that invoke the need of dedicated specialized care for TIA and minor stroke (68).
The link between carotid and coronary
For decades, due to poor data, poor diagnostic methods and a certain vision of atherosclerosis as global vascular disease, carotid arteries (easily accessible with US) with evaluation of intima media thickness (IMT) and plaques have been used as a surrogate of probable concomitant coronary artery disease, especially in primary cardiovascular prevention. Most likely this is not the case, or at least the relationship is far more complicated because it has to do off course with the presence of disease but ultimately it has also a lot to do with activity of the disease. It is quite undisputable that atherosclerosis found in one arterial district, whether it is carotid arteries, coronary arteries, aorta, peripheral, often is associated with atherosclerosis in one or more other districts. The point is that atherosclerosis is a dynamic disease that affects different patients in a different way; it remains stable for years and at some point in time starts progressing or vice-versa; it expresses itself with spikes of instability that we observe in one district and not in another. Therefore, while we understood already several features and mechanisms of natural history of atherosclerosis, we still have poor comprehension of the actual links between what we consider the same disease (or at least a very similar disease) in different districts. The focus is by default concentrated on coronary artery disease and carotid artery disease because they are the more prevalent, morbidly and deadly districts.
The title of an editorial by JACC by Prediman Shah “Can Carotid Plaque Predict Coronary Plaque?” (69), shows the growing interest between the relationship of the carotid and coronary atherosclerosis by exploring the differences and similarities, with the objective of identifying some features that could be used for both organs to modify of risk.
Cohen et al. (70), assessed the relationship between carotid artery disease by ultrasound and coronary artery disease by coronary CT angiography in 150 subjects and they found that the presence of a carotid plaque predicted the presence of any calcified coronary plaque (calcium score >0) with an odds ratio of 5.4 (P<0.0001). Carotid plaque was present in 107 of 150 subjects (71.3%) and of these 107 subjects, 28% had no coronary plaque on calcium scan whereas the coronary plaque was present in 87 of 150 subjects (58%) on calcium scan, and of these 87 subjects, carotid plaque was present in 77 (87.5%).
Sub-clinical atherosclerosis
A challenging field of research and clinical implementation is how to identify subclinical atherosclerosis and its prognostic impact. It is important to underline that even if detecting subclinical atherosclerosis could be important in terms of risk stratification, until recently there was no proof that such detection could be translated into a better outcome (69). In the scenario of imaging detection of subclinical atherosclerosis, the approach is completely different for the analysis of carotid extracranial arteries and coronary arteries.
For carotid artery imaging, probably the most widely accepted and performed method to explore the carotid arteries is conventional US; the obvious methodological advantages of this technique include its totally noninvasive nature, lack of any risk, ease of use, and suitability for repeated imaging. The target that is considered a marker of subclinical atherosclerosis is Intima-Media-Thickness (IMT) that is widely demonstrated to be a strong parameter for the prediction of clinical cardiovascular events (70,71). Moreover, IMT seems to have efficacy in assessing progression and regression of atherosclerosis (72).
Different approach is requested for the coronary arteries because of the use of conventional US is not possible and most of authors suggest the CACS as the reference tool for the identification of subclinical atherosclerosis in coronary arteries (73). However, CACS has some drawbacks including radiation exposure (however lower for CACS that CT angiography of coronary arteries) and missing subjects with non-calcified plaque.
The latest guidelines on Guideline on the Management of Blood Cholesterol from the American Heart Association (AHA) and American College of Cardiology (ACC) (74), stated for the first time in an actual official guideline that: (I) In individuals 40–75 years of age without DM and with LDL-C levels ≥70 mg/dL with risk status uncertain, consider using CACS to improve specificity; (II) In individuals 40–75 years of age without DM and with LDL-C levels ≥70 mg/dL, with 10-year ASCVD risk 7.5–19.9%, if a decision about statin therapy is uncertain, consider measuring CACS. If CACS is 0, treatment with statin therapy may be withheld or delayed, except in cigarette smokers, those with diabetes mellitus, and those with a strong family history of premature ASCVD. A CACS score of 1 to 99 favors statin therapy, especially in those ≥55 years of age. For any patient, if the CACS score is ≥100 Agatston units or ≥75th percentile, statin therapy is indicated unless otherwise deferred by the outcome of clinician-patient risk discussion (Grundy SM et al. JACC 2018). This guideline introduces CACS as a decision-making tool for modulating statin therapy.
In 2018, the SCOT-HEART (75) trial with 5-year follow-up results was also published showing, probably for the first time in the history of medicine, that the use of Coronary CT Angiography in addition to standard care in patients with stable chest pain resulted in a significantly lower rate of death from coronary heart disease or nonfatal myocardial infarction at 5 years than standard care alone, without resulting in a significantly higher rate of coronary angiography or coronary revascularization (SCOT-HEART, NEJM 2018). This might seem something unrelated to subclinical atherosclerosis. Instead, what we could define as the “pleiotropic” effect of CT was more evident in the population of patient with chest pain and non significant obstructive disease. In fact, in this population the evidence of coronary atherosclerosis led referring physicians to address more aggressively primary prevention strategies and the patients to adhere more obediently to physicians’ prescriptions. In this study there was no indication given to patients or referring physicians concerning the actions to be taken based on the results of the diagnostic results.
This study and other multicenter registries such as the CONFIRM (76), in which it has been demonstrated that statin are able to improve prognosis in patients with non obstructive CAD while they have no effect on the prognosis of patients with completely normal coronary arteries, support the idea of a imaging guided optimal medical therapy which falls in the field of the so-called individualized/personalized medicine.
Imaging strategies and future options
An in-depth analysis of the plaque features that should be classified as protective or counter-protective will be the main target of cardiovascular imaging in the next years. In particular, with the advances of knowledge in the biological processes together with evolution in the field of imaging technologies it will be possible to perform a detailed and tailored risk stratification with therapeutic implications.
As we previously showed the presence of calcium has different value in carotid and coronary arteries, with protective effect in the first and increased risk of cardio/cerebrovascular event for the second. However, it seems there are 2 types of calcium with a completely opposite risk profile. It is possible that in the near future it would be possible to distinguish between these 2 types of calcifications. The recent introduction of dual energy CT scanners could offer several advantages in the explanation of the different profile of risk in carotid and coronary arteries calcified plaques by distinguishing between hydroxyapatite and calcium oxalate calcifications (and their different profile of risk) thanks to spectral analysis. Stabilizing the unstable plaque is a key goal in reducing the risk of MI or IS. PET, MR and CT and the so-called molecular applications that are still in early phases of development and validation; all 3 modalities (surely PET is more advanced in this field) may become able to explore biomarkers of plaque activity and stability directly in the coronary/carotid artery wall. The development of these probes would allow for not only identification of at-risk atheroma but also permit clinicians to assess response to medical therapy (statins, anti-inflammatory drugs).
As a parallel line of research is worth to mention the assessment of epicardial fat in the cardiac field. This assessment is quite simple to perform and there are conflicting evidence on its real potential. More recently it has been advocated that the assessment of peri-coronary fat might be a surrogate of vessel wall inflammation as detected and measure on CCT (77).
The natural history of atherosclerosis is considered to be a dynamic process varying from early lesion development to more advanced plaques complicated by acute thrombosis. In addition, it is not sure nor obvious that coronary atherosclerosis and carotid atherosclerosis follow parallel natural histories.
Current guidelines for primary prevention lend support to the use of statins to treat increased cholesterol in individuals who are at increased risk based on conventional epidemiological risk scoring systems; however, future guidelines might incorporate the recommendation for statin treatment in patients with normal cholesterol who are at increased risk because of another risk factor or biomarker. In fact, nowadays, the Framingham risk score, as well as the other epidemiologically derived risk scores, although useful in characterizing risk in groups of subjects, leave considerable uncertainty, especially in those in the low- to intermediate-risk (69).
The monitoring of plaque features related to the increased risk of stroke could represent a critical step to verify if the patient continues to belong to a risk class or after therapies there is a variation in the class risk. It is well known that therapeutic lifestyle modification, medical therapy to control risk factors significantly reduce atherothrombotic cardiovascular events (69).
Summary and conclusions
Imaging, currently, plays already an important role in cardiovascular risk stratification. Thanks to the increasing potentialities of intravascular and extravascular imaging methods venues are now open to the clinicians and not only the identification of the features of plaque vulnerability but the monitoring of plaque’s response to the drug therapy is possible.
This represent a critical step to improving the management of subjects with atherosclerotic plaque, by checking the condition and structure of the plaque and by identifying condition susceptible to reversion of with a risk of rupture.
Acknowledgments
Funding: None.
Footnote
Provenance and Peer Review: This article was commissioned by the Guest Editor (Luca Saba) for the series “Advanced Imaging in the diagnosis of Cardiovascular Diseases” published in Cardiovascular Diagnosis and Therapy. The article was sent for external peer review organized by the Guest Editor and the editorial office.
Conflicts of Interest: All authors have completed the ICMJE uniform disclosure form (available at http://dx.doi.org/10.21037/cdt-20-528). The series “Advanced Imaging in the diagnosis of Cardiovascular Diseases” was commissioned by the editorial office without any funding or sponsorship. FC serves as an unpaid editorial board member of Cardiovascular Diagnosis and Therapy from Jul 2019 to Jun 2021. LS served as the unpaid Guest Editor of the series and serves as an unpaid editorial board member of Cardiovascular Diagnosis and Therapy from Jul 2019 to Jun 2021. The authors have no other conflicts of interest to declare.
Ethical Statement: The authors are accountable for all aspects of the work in ensuring that questions related to the accuracy or integrity of any part of the work are appropriately investigated and resolved.
Open Access Statement: This is an Open Access article distributed in accordance with the Creative Commons Attribution-NonCommercial-NoDerivs 4.0 International License (CC BY-NC-ND 4.0), which permits the non-commercial replication and distribution of the article with the strict proviso that no changes or edits are made and the original work is properly cited (including links to both the formal publication through the relevant DOI and the license). See: https://creativecommons.org/licenses/by-nc-nd/4.0/.
References
- Yanez ND, Burke GL, Manolio T, et al. CHS Collaborative Research Group. Sibling history of myocardial infarction or stroke and risk of cardiovascular disease in the elderly: the Cardiovascular Health Study. Ann Epidemiol 2009;19:858-66. [Crossref] [PubMed]
- Naghavi M, Libby P, Falk E, et al. From vulnerable plaque to vulnerable patient: a call for new definitions and risk assessment strategies: Part II. Circulation 2003;108:1772-8. [Crossref] [PubMed]
- Redgrave JN, Lovett JK, Gallagher PJ, et al. Histological assessment of 526 symptomatic carotid plaques in relation to the nature and timing of ischemic symptoms: The Oxford plaque study. Circulation 2006;113:2320-8. [Crossref] [PubMed]
- Hayashi T, Kiyoshima T, Matsuura M, et al. Plaque erosion in the culprit lesion is prone to develop a smaller myocardial infarction size compared with plaque rupture. Am Heart J 2005;149:284-90. [Crossref] [PubMed]
- Kolodgie FD, Gold HK, Burke AP, et al. Intraplaque hemorrhage and progression of coronary atheroma. N Engl J Med 2003;349:2316-25. [Crossref] [PubMed]
- Naylor AR, Sillesen H, Schroeder TV. Clinical and imaging features associated with an increased risk of early and late stroke in patients with symptomatic carotid disease. Eur J Vasc Endovasc Surg 2015;49:513-23. [Crossref] [PubMed]
- Saba L, Yuan C, Hatsukami TS, et al. Vessel Wall Imaging Study Group of the American Society of Neuroradiology. Carotid Artery Wall Imaging: Perspective and guidelines from the ASNR Vessel Wall Imaging Study Group and Expert Consensus Recommendations of the American Society of Neuroradiology. AJNR Am J Neuroradiol 2018;39:E9-E31. [Crossref] [PubMed]
- Schoenhagen P, Stone GW, Nissen SE, et al. Coronary plaque morphology and frequency of ulceration distant from culprit lesions in patients with unstable and stable presentation. Arterioscler Thromb Vasc Biol 2003;23:1895-900. [Crossref] [PubMed]
- Goldstein JA, Demetriou D, Grines CL, et al. Multiple complex coronary plaques in patients with acute myocardial infarction. N Engl J Med 2000;343:915-22. [Crossref] [PubMed]
- de Weert TT, Cretier S, Groen HC, et al. Atherosclerotic plaque surface morphology in the carotid bifurcation assessed with multidetector computed tomography angiography. Stroke 2009;40:1334-40. [Crossref] [PubMed]
- Rothwell PM, Eliasziw M, Gutnikov SA, et al. Analysis of pooled data from the randomised controlled trials of endarterectomy for symptomatic carotid stenosis. Lancet 2003;361:107-16. [Crossref] [PubMed]
- Stone GW, Maehara A, Lansky AJ, et al. PROSPECT Investigators. A prospective natural-history study of coronary atherosclerosis. N Engl J Med 2011;364:226-35. [Crossref] [PubMed]
- Barnett HJ, Taylor DW, Eliasziw M, et al. Benefit of carotid endarterectomy in patients with symptomatic moderate or severe stenosis. North American Symptomatic Carotid Endarterectomy Trial Collaborators. N Engl J Med 1998;339:1415-25. [Crossref] [PubMed]
- Prabhakaran S, Rundek T, Ramas R, et al. Carotid plaque surface irregularity predicts ischemic stroke: the northern Manhattan study. Stroke 2006;37:2696-701. [Crossref] [PubMed]
- Troyer A, Saloner D, Pan XM, et al. Assessment of Carotid Stenosis by Comparison with Endarterectomy Plaque Trial Investigators. Major carotid plaque surface irregularities correlate with neurologic symptoms. J Vasc Surg 2002;35:741-7. [Crossref] [PubMed]
- Sinclair H, Bourantas C, Bagnall A, et al. OCT for the identification of vulnerable plaque in acute coronary syndrome. JACC Cardiovasc Imaging 2015;8:198-209. [Crossref] [PubMed]
- Gogas BD, Farooq V, Serruys PW, et al. Assessment of coronary atherosclerosis by IVUS and IVUS-based imaging modalities: progression and regression studies, tissue composition and beyond. Int J Cardiovasc Imaging 2011;27:225-37. [Crossref] [PubMed]
- Randoux B, Marro B, Koskas F, et al. Carotid artery stenosis: prospective comparison of CT, three-dimensional gadolinium-enhanced MR, and conventional angiography. Radiology 2001;220:179-85. [Crossref] [PubMed]
- Kanber B, Hartshorne TC, Horsfield MA, et al. Quantitative assessment of carotid plaque surface irregularities and correlation to cerebrovascular symptoms. Cardiovasc Ultrasound 2013;11:38. [Crossref] [PubMed]
- Agatston AS, Janowitz WR, Hildner FJ, et al. Quantification of coronary artery calcium using ultrafast computed tomography. J Am Coll Cardiol 1990;15:827-32. [Crossref] [PubMed]
- Powers WJ, Rabinstein AA, Ackerson T, et al. American Heart Association Stroke Council. 2018 Guidelines for the Early Management of Patients With Acute Ischemic Stroke: A Guideline for Healthcare Professionals From the American Heart Association/American Stroke Association. Stroke 2018;49:e46-e110. [Crossref] [PubMed]
- Saba L, Caddeo G, Sanfilippo R, et al. CT and ultrasound in the study of ulcerated carotid plaque compared with surgical results: potentialities and advantages of multidetector row CT angiography. AJNR Am J Neuroradiol 2007;28:1061-6. [Crossref] [PubMed]
- Saba L, Caddeo G, Sanfilippo R, et al. Efficacy and Sensitivity of axial scans and different reconstruction methods in the study of the ulcerated carotid plaque by using multi-detector-row CT angiography. Comparison with surgical results. AJNR Am J Neuroradiol 2007;28:716-23. [Crossref] [PubMed]
- Ridolfi RL, Hutchins GM. The relationship between coronary artery lesions and myocardial infarcts: ulceration of atherosclerotic plaques precipitating coronary thrombosis. Am Heart J 1977;93:468-86. [Crossref] [PubMed]
- Obaid DR, Calvert PA, Brown A, et al. Coronary CT angiography features of ruptured and high-risk atherosclerotic plaques: Correlation with intra-vascular ultrasound. J Cardiovasc Comput Tomogr 2017;11:455-61. [Crossref] [PubMed]
- Dubois CL, Dens JA. Coronary ulcer. Heart 2005;91:222. [Crossref] [PubMed]
- Ratiu M, Chitu M, Benedek I, et al. Impact of coronary plaque geometry on plaque vulnerability and its association with the risk of future cardiovascular events in patients with chest pain undergoing coronary computed tomographic angiography-the GEOMETRY study: Protocol for a prospective clinical trial. Medicine (Baltimore) 2018;97:e13498. [Crossref] [PubMed]
- Wartman WB. Occlusion of the coronary arteries by hemorrhage into their walls. Am Heart J 1938;15:459-70. [Crossref]
- Otsuka F, Sakakura K, Yahagi K, et al. Has our understanding of calcification in human coronary atherosclerosis progressed? Arterioscler Thromb Vasc Biol 2014;34:724-36. Erratum in: Arterioscler Thromb Vasc Biol 2014;34:e17. [Crossref] [PubMed]
- Janowitz WR, Agatston AS, Viamonte M Jr. Comparison of serial quantitative evaluation of calcified coronary artery plaque by ultrafast computed tomography in persons with and without obstructive coronary artery disease. Am J Cardiol 1991;68:1-6. [Crossref] [PubMed]
- Budoff MJ, Achenbach S, Blumenthal RS, et al. Assessment of coronary artery disease by cardiac computed tomography: a scientific statement from the American Heart Association Committee on Cardiovascular Imaging and Intervention, Council on Cardiovascular Radiology and Intervention, and Committee on Cardiac Imaging, Council on Clinical Cardiology. Circulation 2006;114:1761-91. [Crossref] [PubMed]
- Detrano R, Guerci AD, Carr JJ, et al. Coronary calcium as a predictor of coronary events in four racial or ethnic groups. N Engl J Med 2008;358:1336-45. [Crossref] [PubMed]
- Stefanadis C, Antoniou CK, Tsiachris D, et al. Coronary Atherosclerotic Vulnerable Plaque: Current Perspectives. J Am Heart Assoc 2017;6:e005543. [Crossref] [PubMed]
- Nandalur KR, Hardie AD, Raghavan P, et al. Composition of the stable carotid plaque: insights from a multidetector computed tomography study of plaque volume. Stroke 2007;38:935-40. [Crossref] [PubMed]
- Nandalur KR, Baskurt E, Hagspiel KD, et al. Calcified carotid atherosclerotic plaque is associated less with ischemic symptoms than is noncalcified plaque on MDCT. AJR Am J Roentgenol 2005;184:295-8. [Crossref] [PubMed]
- Vasuri F, Fittipaldi S, Pini R, et al. Diffuse calcifications protect carotid plaques regardless of the amount of neoangiogenesis and related histological complications. Biomed Res Int 2015;2015:795672.
- Baradaran H, Al-Dasuqi K, Knight-Greenfield A, et al. Association between Carotid Plaque Features on CTA and Cerebrovascular Ischemia: A Systematic Review and Meta-Analysis. AJNR Am J Neuroradiol 2017;38:2321-6. [Crossref] [PubMed]
- Katano H, Nishikawa Y, Yamada H, et al. Differential Expression of microRNAs in Severely Calcified Carotid Plaques. J Stroke Cerebrovasc Dis 2018;27:108-17. [Crossref] [PubMed]
- Bischetti S, Scimeca M, Bonanno E, et al. Carotid plaque instability is not related to quantity but to elemental composition of calcification. Nutr Metab Cardiovasc Dis 2017;27:768-74. [Crossref] [PubMed]
- Felton CV, Crook D, Davies MJ, et al. Relation of plaque lipid composition and morphology to the stability of human aortic plaques. Arterioscler Thromb Vasc Biol 1997;17:1337-45. [Crossref] [PubMed]
- Xu D, Hippe DS, Underhill HR, et al. Prediction of high-risk plaque development and plaque progression with the carotid atherosclerosis score. JACC Cardiovasc Imaging 2014;7:366-73. [Crossref] [PubMed]
- Trelles M, Eberhardt KM, Buchholz M, et al. CTA for screening of complicated atherosclerotic carotid plaque--American Heart Association type VI lesions as defined by MRI. AJNR Am J Neuroradiol 2013;34:2331-7. [Crossref] [PubMed]
- Kanaya T, Noguchi T, Otsuka F, et al. Optical coherence tomography-verified morphological correlates of high-intensity coronary plaques on non-contrast T1-weighted magnetic resonance imaging in patients with stable coronary artery disease. Eur Heart J Cardiovasc Imaging 2019;20:75-83. [Crossref] [PubMed]
- Grønholdt ML, Wiebe BM, Laursen H, et al. Lipid-rich carotid artery plaques appear echolucent on ultrasound B-mode images and may be associated with intraplaque haemorrhage. Eur J Vasc Endovasc Surg 1997;14:439-45. [Crossref] [PubMed]
- Noritomi T, Sigel B, Swami V, et al. Carotid plaque typing by multiple-parameter ultrasonic tissue characterization. Ultrasound Med Biol 1997;23:643-50. [Crossref] [PubMed]
- Kockx MM, Cromheeke KM, Knaapen MW, et al. Phagocytosis and macrophage activation associated with hemorrhagic microvessels in human atherosclerosis. Arterioscler Thromb Vasc Biol 2003;23:440-6. [Crossref] [PubMed]
- Brinjikji W, Lehman VT, Huston J 3rd, et al. The association between carotid intraplaque hemorrhage and outcomes of carotid stenting: a systematic review and meta-analysis. J Neurointerv Surg 2017;9:837-42. [Crossref] [PubMed]
- Saam T, Hetterich H, Hoffmann V, et al. Meta-analysis and systematic review of the predictive value of carotid plaque hemorrhage on cerebrovascular events by magnetic resonance imaging. J Am Coll Cardiol 2013;62:1081-91. [Crossref] [PubMed]
- Narumi S, Sasaki M, Ohba H, et al. Prediction of carotid plaque characteristics using non-gated MR imaging: correlation with endarterectomy specimens. AJNR Am J Neuroradiol 2013;34:191-7. [Crossref] [PubMed]
- Wintermark M, Jawadi SS, Rapp JH, et al. High-resolution CT imaging of carotid artery atherosclerotic plaques. AJNR Am J Neuroradiol 2008;29:875-82. [Crossref] [PubMed]
- Saba L, Francone M, Bassareo PP, et al. CT Attenuation Analysis of Carotid Intraplaque Hemorrhage. AJNR Am J Neuroradiol 2018;39:131-7. [Crossref] [PubMed]
- Htun NM, Chen YC, Lim B, et al. Near-infrared autofluorescence induced by intraplaque hemorrhage and heme degradation as marker for high-risk atherosclerotic plaques. Nat Commun 2017;8:75. [Crossref] [PubMed]
- Burke AP, Farb A, Malcom GT, et al. Plaque rupture and sudden death related to exertion in men with coronary artery disease. JAMA 1999;281:921-6. [Crossref] [PubMed]
- Barger AC, Beeuwkes R III, Lainey LL, et al. Hypothesis: vasa vasorum and neovascularization of human coronary arteries: a possible role in the pathophysiology of atherosclerosis. N Engl J Med 1984;310:175-7. [Crossref] [PubMed]
- McCarthy MJ, Loftus IM, Thompson MM, et al. Angiogenesis and the atherosclerotic carotid plaque: an association between symptomatology and plaque morphology. J Vasc Surg 1999;30:261-8. [Crossref] [PubMed]
- Mofidi R, Crotty TB, McCarthy P, et al. Association between plaque instability, angiogenesis and symptomatic carotid occlusive disease. Br J Surg 2001;88:945-50. [Crossref] [PubMed]
- Huang R, Abdelmoneim SS, Ball CA, et al. Detection of Carotid Atherosclerotic Plaque Neovascularization Using Contrast Enhanced Ultrasound: A Systematic Review and Meta-Analysis of Diagnostic Accuracy Studies. J Am Soc Echocardiogr 2016;29:491-502. [Crossref] [PubMed]
- Shimada Y, Oikawa K, Fujiwara S, et al. Comparison of Three-Dimensional T1-Weighted Magnetic Resonance and Contrast-Enhanced Ultrasound Plaque Images for Severe Stenosis of the Cervical Carotid Artery. J Stroke Cerebrovasc Dis 2017;26:1916-22. [Crossref] [PubMed]
- Saba L, Lai ML, Montisci R, et al. Association between carotid plaque enhancement shown by multidetector CT angiography and histologically validated microvessel density. Eur Radiol 2012;22:2237-45. [Crossref] [PubMed]
- Romero JM, Babiarz LS, Forero NP, et al. Arterial wall enhancement overlying carotid plaque on CT angiography correlates with symptoms in patients with high grade stenosis. Stroke 2009;40:1894-6. [Crossref] [PubMed]
- Millon A, Boussel L, Brevet M, et al. Clinical and histological significance of gadolinium enhancement in carotid atherosclerotic plaque. Stroke 2012;43:3023-8. [Crossref] [PubMed]
- Amano H, Koizumi M, Okubo R, et al. Comparison of Coronary Intimal Plaques by Optical Coherence Tomography in Arteries With Versus Without Internal Running Vasa Vasorum. Am J Cardiol 2017;119:1512-7. [Crossref] [PubMed]
- Verjans JW, Osborn EA, Ughi GJ, et al. Targeted Near-Infrared Fluorescence Imaging of Atherosclerosis: Clinical and Intracoronary Evaluation of Indocyanine Green. JACC Cardiovasc Imaging 2016;9:1087-95. [Crossref] [PubMed]
- Vergallo R, Xing L, Minami Y, et al. Associations between the Framingham Risk Score and coronary plaque characteristics as assessed by three-vessel optical coherence tomography. Coron Artery Dis 2016;27:460-6. [Crossref] [PubMed]
- Amarenco P, Lavallée PC, Labreuche J, et al. One-year risk of stroke after transient ischemic attack or minor stroke. N Engl J Med 2016;374:1533-42. [Crossref] [PubMed]
- Kim AS, Easton JD, Johnston SC. Risk of Stroke after Transient Ischemic Attack or Minor Stroke. N Engl J Med 2016;375:386-7. [Crossref] [PubMed]
- Mijalski C, Silver B. TIA Management: Should TIA Patients be Admitted? Should TIA Patients Get Combination Antiplatelet Therapy? Neurohospitalist 2015;5:151-60. [Crossref] [PubMed]
- Sacco RL, Rundek T. The Value of Urgent Specialized Care for TIA and Minor Stroke. N Engl J Med 2016;374:1577-9. [Crossref] [PubMed]
- Shah PK. Can carotid plaque predict coronary plaque? JACC Cardiovasc Imaging 2013;6:1168-71. [Crossref] [PubMed]
- Cohen GI, Aboufakher R, Bess R, et al. Relationship between carotid disease on ultrasound and coronary disease on CT angiography. JACC Cardiovasc Imaging 2013;6:1160-7. [Crossref] [PubMed]
- Lorenz MW, Polak JF, Kavousi M, et al. PROG-IMT Study Group. Carotid intima-media thickness progression to predict cardiovascular events in the general population (the PROG-IMT collaborative project): a meta-analysis of individual participant data. Lancet 2012;379:2053-62. [Crossref] [PubMed]
- Lorenz MW, Markus HS, Bots ML, et al. Prediction of clinical cardiovascular events with carotid intima-media thickness: a systematic review and meta-analysis. Circulation 2007;115:459-67. [Crossref] [PubMed]
- Stanford W. Coronary artery calcification as an indicator of preclinical coronary artery disease. Radiographics 1999;19:1409-19. [Crossref] [PubMed]
- Grundy SM, Stone NJ, Bailey AL, et al. 2018 AHA/ACC/AACVPR/AAPA/ABC/ACPM/ADA/AGS/APhA/ASPC/NLA/PCNA Guideline on the Management of Blood Cholesterol: A Report of the American College of Cardiology/American Heart Association Task Force on Clinical Practice Guidelines. J Am Coll Cardiol 2019;73:e285-e350. [Crossref] [PubMed]
- SCOT-HEART Investigators, Newby DE, Adamson PD, et al. Coronary CT Angiography and 5-Year Risk of Myocardial Infarction. N Engl J Med 2018;379:924-33. [Crossref] [PubMed]
- Xie JX, Eshtehardi P, Varghese T, et al. Prognostic Significance of Nonobstructive Left Main Coronary Artery Disease in Women Versus Men: Long-Term Outcomes From the CONFIRM (Coronary CT Angiography Evaluation For Clinical Outcomes: An International Multicenter) Registry. Circ Cardiovasc Imaging 2017;10:e006246. [Crossref] [PubMed]
- Oikonomou EK, Williams MC, Kotanidis CP, et al. A novel machine learning-derived radiotranscriptomic signature of perivascular fat improves cardiac risk prediction using coronary CT angiography. Eur Heart J 2019;40:3529-43. [Crossref] [PubMed]