Congenital pulmonary vascular anomalies
Introduction
Congenital pulmonary artery anomalies are, by definition, present at birth however; age at clinical presentation is variable depending on restriction of blood flow or plethora of flow to the pulmonary arteries. Most congenital pulmonary artery abnormalities are associated with congenital heart disease and may be related to underlying syndromes. Transthoracic echocardiography (TTE) remains an essential tool in the evaluation of these conditions however; magnetic resonance imaging (MRI) and computed tomography angiography (CTA) have emerged as valuable, complimentary tools. Modern CT scanners with improved detectors, faster gantry rotation time and increased pitch have yielded improved resolution, and decreased exposure time to allow for rapid scan times, between 0.2 and 0.4 seconds. This rapid scan time allows for a reduced radiation dose while minimizing artifact secondary to respiratory motion. Rapid scanning provides high quality images for preoperative or pre-catheterization evaluation, usually without the need for sedation or breath holds, which is very important in infants and young children.
CTA scan technique
Patient preparation and attention to technique are critical to obtaining a diagnostic quality CTA. Both site and size of intravenous (IV) access are important. Anomalous or post-operative venous anatomy should be considered prior to selection of IV site. Injection rate and duration of contrast material injection can be manipulated to ensure optimal opacification of the vessels of interest. A fast injection rate will deliver a tight bolus concomitantly increasing the magnitude of enhancement. For older children, IV line larger than a 20 gauge is recommended for power injection, or a power injectable central venous catheter. Maximum flow recommendations are listed in Table 1. It is important to remember that patient-related factors also affect the magnitude of vascular contrast enhancement, especially patient weight (1). Smaller patients have a smaller blood volume and therefore, have less dilution of a given volume of contrast material. This results in higher (more dense) vascular enhancement. The volume of available contrast material becomes a factor in small infants. A dose of 3 mL/kg is utilized for infants under 5 kg and 2 mL/kg for all other patients. For a 2 kg infant the dose of intravenous contrast material is 6 mL. This small volume may actually remain primarily in the injection tubing. The contrast material can be diluted up to a 50% contrast/saline solution in order to increase the volume to 12 mL. In addition, a dual injection method, with a saline bolus, will also increase the volume of contrast material available for intravascular opacification. Optimal contrast enhancement is achieved with dilute contrast material, especially given the lower intravascular blood volume of the infant. Non dilute contrast material has the disadvantage of both lower overall volume for injection and significant high density which can cause streak artifact and increased overall radiation dose due to density. Bolus tracking is used to trigger scan initiation. Typically, when there is concern for pulmonary arterial abnormalities, the region of interest should be placed on the pulmonary arteries however, knowledge of cardiac abnormalities and admixing from the left and right heart before scanning is necessary to reduce errors that may occur due to other cardiac defects. The upper abdomen should be included in all patients to assess abdominal situs and evaluate for abnormal arterial supply or venous drainage below the diaphragm.
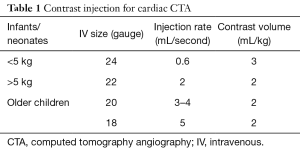
Full table
Congenital pulmonary arterial anomalies
The development of the pulmonary arteries occurs concomitant with the development of the aortic arch after the separation of the embryonic truncus. Utilizing the totipotent aortic arch model, anomalies of the formation of the arch and pulmonary arteries can be explained by faulty regression of various components of the embryonic double arches, occurring on either the right or left side (Figure 1).
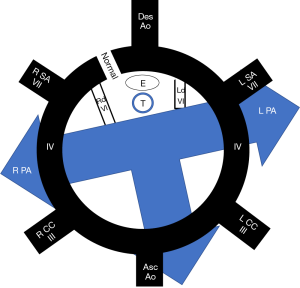
Pulmonary sling
Isolated anatomic abnormalities of the pulmonary arteries are uncommon. The pulmonary arteries are derived during embryogenesis from the 6th aortic arch, also called the pulmonary arch. During embryogenesis, this 6th pulmonary arch arises as an early capillary plexus growing in association with early tracheal and lung buds. The left sided pulmonary arch typically forms a major channel connecting the left pulmonary artery to the aorta, the ductus arteriosus. The ductus arteriosus is important during fetal development allowing more oxygen rich blood from the umbilical vein to bypass the developing lungs and flow directly to the aorta. Anomalies that arise from the abnormal formation of this 6th pulmonary arch include the aberrant origin of the left pulmonary artery from the right pulmonary artery, the pulmonary sling (Figure 2). The anomalous course extends from the posterior wall of the right pulmonary artery crossing to the left, posterior to the trachea and anterior to the esophagus. Along with the abnormal vascular course, tracheobronchial abnormalities may have a greater impact on prognosis (2). Approximately one third of infants have impingement of the right main stem bronchus although otherwise, a normal airway and location of the carina (type I). A more severe form (type II) however, can be associated with a low lying carina and long segment tracheal stenosis. Tracheal stenosis may be related to compression or associated with complete cartilaginous tracheal rings. Anomalies of the right sided bronchi are also common with a tracheal bronchus seen in both types. The typical presentation of these rare anomalies is an infant with stridor although there are rare reports of a pulmonary sling being discovered as an asymptomatic lesion in adults (3). Associated intracardiac abnormalities are also common and were reported in 85% of patients by Xie et al. (4). The lobar anatomy of the lung, anomalies of right bronchial branching, anomalies of right pulmonary artery branching and degree of compression are well evaluated with CT angiography. All of these are important considerations prior to surgical correction of these lesions.
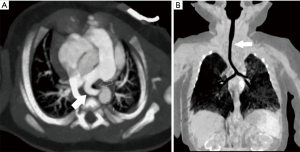
Pulmonary valve stenosis
Pulmonary valve disease can range from isolated valvar stenosis to complete atresia. Time of presentation varies by severity of stenosis, with critical pulmonary stenosis or atresia presenting in the newborn period. For patients with isolated valvar stenosis, balloon valvuloplasty alone is the treatment of choice and is indicated when the right ventricular to pulmonary artery peak to peak gradient exceeds 50 mmHg with fairly durable results. A recent report by Devanagondi et al., demonstrated long term cumulative incidence of development of moderate pulmonary regurgitation (PR) in over 60% of patients undergoing balloon valvuloplasty, although progression to valve replacement was uncommon. Critical PS, low BSA, small pulmonary annulus size and younger age were associated with the development of PR (5).
Pulmonary atresia (PA)
PA is typically described in relationship to the presence of an intact (PA-IVS) or absent ventricular septum (PA-VSD). PA may be at the tricuspid valve plane, involve the main pulmonary artery or involve interruption of the right or left pulmonary artery proximally or at the hilum. The presence of a VSD allows for blood from the right heart to flow across the septum, out the aorta through a patent ductus arteriosus (PDA) to the lungs, and thus relatively normal sized branch pulmonary arteries. An intact septum requires another path for blood to reach the left heart and lungs, typically through the foramen ovale with resultant hypoplasia of the right ventricle. In patients with PA/VSD, major aortopulmonary collateral arteries (MAPCAs) are typically present and native pulmonary arteries are generally hypoplastic or absent. In both lesions, pulmonary blood flow is via the systemic circulation either via the PDA or from MAPCAs. Urgent early interventions may be required in order to ensure that the PDA remains patent when this is the primary source of pulmonary blood flow. Typically, intravenous prostaglandin therapy will be initiated immediately until a more definitive mechanism to provide pulmonary blood flow is established (6).
PDA stenting
In patients in whom pulmonary blood flow is ductal dependent, intravenous prostaglandin infusion is required until a stable source of pulmonary blood flow is established. Traditionally, blood flow to the pulmonary arteries was established by surgical creation of a systemic to pulmonary shunt. With the advent of micro catheters and advance coronary stent technology, stent dilation has been gaining popularity regardless of ductal morphology as it avoids a neonatal cardiopulmonary bypass procedure and blood product exposure and allows a shorter intubation time and ICU stay (6-11).
The approach to PDA stenting is complex. Factors that determine the approach include patient size, additional congenital abnormalities, tortuosity of the PDA and its origin from the aorta. In most patients, access via the carotid arteries provides the most direct route and in infants, the largest artery for access. Carotid access can be achieved percutaneously or via surgical cut down. Additional access sites include axillary or subclavian artery and femoral artery. Approach is dependent on operator experience and comfort level with each access site, as well as the availability of surgical assistance. Pre-procedural 3-dimensional imaging is very helpful if the PDA cannot be fully delineated via echocardiogram.
From a technical standpoint, if access is planned from the carotid artery, inverting the patient on the catheterization table is quite helpful as the catheterization equipment can be difficult to manage without the support of a table due to length and the extremely short working area within the body. Imaging is performed via the sheath or angiographic catheter to assess the origin and insertion. Ideally, ductal constriction would be present to avoid stent embolization. Discontinuation of prostaglandin a few hours prior to the procedure can be helpful to encourage this. Determination of stent size and length is based on ductal anatomy. It is very important to cover both the origin and insertion with stent as areas left uncovered are likely to constrict following discontinuation of prostaglandin, thus the stent selected should be slightly longer than the actual PDA (7,8). This can be difficult to determine in very tortuous PDAs as they tend to elongate with placement of a stiff wire. The operator should have a full array of bare metal coronary or other small diameter stents available as one should anticipate ductal spasm with placement of a stiffer interventional wire/catheter across the PDA. We elect to keep prostaglandin infusions in line as well as bolus doses should prolonged spasm occur resulting in profound desaturation. Once the stent length is determined, the diameter can be determined. For smaller stent lengths (15 and 18 mm) in a newborn infant, a 3.5-mm diameter is usually sufficient. With longer distances (23 mm) consideration should be given to increasing the diameter to at least 4 mm to account for resistance. Just as with surgical shunts, consideration must be given to achieving adequate pulmonary blood flow without depriving the body of adequate circulation.
The PDA stent must extend into the main pulmonary artery or branch PA (depending on anatomy) if the insertion is to be adequately covered (Figure 3). Care should be taken to avoid significant extension of the stent into the branch pulmonary arteries if possible to minimize the degree of surgical arterioplasty that will be required in the future. Many times, protrusion is unavoidable but rarely results in significant compromise of flow to the opposite pulmonary artery despite jailing of the orifice (12).
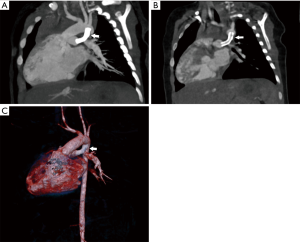
MAPCAs
PA-VSD with MAPCAs is a rare anomaly occurring in approximately 10/100,000 live births (12). Most will present in infancy with severe cyanosis, with heart failure seen in approximately one quarter of patients. In patients with well-balanced circulation, presentation in early adulthood has been described (13). PA-VSD with MAPCAs is the most severe form of tetralogy of Fallot (TOF). TTE and cardiac catheterization with direct injection into each collateral vessel with indirect imaging of the native pulmonary arteries historically were the primary imaging modalities in these patients however; advances in cardiac CT imaging has led to a shift away from invasive imaging for delineation of collateral supply and demonstration of the native pulmonary arteries if present (Figure 4) (14). Surgical planning can now be facilitated with the global imaging made possible by noninvasive cardiac CT. In addition to the delineation of cardiac structural abnormalities, CT allows the visualization and optimal sizing of the native pulmonary arteries as well as localization and sizing of any MAPCAs. Whereas TTE has a limited acoustic window for the evaluation of the branch pulmonary arteries, on CTA, segmental pulmonary blood flow can be depicted clearly and is necessary for surgical planning. In a recent report by Liu et al., imaging data in 116 patients with PA and VSD, CTA was shown to be more accurate than both catheterization and TTE for MAPCA and for the identification of native pulmonary arteries (15). CT evaluation of MAPCAs should entail a precise description of location of origin, size, and presence of origin stenosis, lung segment supplied as well as the anatomic relationship to adjacent structures such as the carina, esophagus and bronchi. Pulmonary blood flow through MAPCAs is by definition at systemic pressures and can lead to over-circulation and heart failure in the absence of stenosis. Repair is accomplished by unifocalization of MAPCAs in conjunction with translocation of the native pulmonary arteries with placement of a Blalock-Taussig shunt from the subclavian artery to the pulmonary arteries or conduit from the right ventricle to pulmonary artery. Following palliation, embolization of MAPCAs is often necessary to decrease the amount of systemic arterial flow to the pulmonary artery bed if dual supply is present and concomitantly reduce the amount of pulmonary hypertension. Complete knowledge of the pulmonary artery anatomy and the confluence of the left and right pulmonary arteries are therefore imperative for surgical planning.
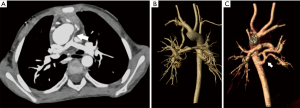
Pulmonary artery stenosis
Native pulmonary artery stenosis is a rare form of congenital heart disease in a variety of patients. Clinically significant native pulmonary stenosis is often found in association with genetic syndromes such as Alagille and Williams syndromes, while Noonan syndrome is typically associated with stenosis of the pulmonary valve and main pulmonary artery which is typically dealt with surgically (16,17). The branch pulmonary artery stenosis in Williams syndrome (Figure 5) typically improves with time and therefore close observation is warranted as stent dilation and angioplasty are often associated with treatment failure due to the arteriopathy of Williams syndrome (18). Intervention should be reserved for patients with severe branch pulmonary artery stenosis that is not improving and resulting in right ventricular hypertension. This may be best accomplished by surgical reconstruction, particularly in younger patients (16). Alagille patients have experienced a bit more success with trans catheter intervention, although numbers and data remain sparse (19). The remainder of pulmonary artery stenosis tends to be post-operative or in association with other complex congenital heart disease. Determining when and if to intervene on pulmonary artery stenosis in the catheterization laboratory is highly dependent on the etiology and location of the stenosis, likelihood of response to trans catheter intervention and patient size. Patient selection is critical to a successful procedure. Angioplasty alone can be successful in isolated proximal lesions (Figure 6). Ostial lesions, compliant lesions and long segment stenoses tend to respond more favorably to stent implantation to maintain vessel patency (20). Stent selection is limited by vessel size and length often resulting in intentional jailing of side branches in smaller children to achieve adequate results. Additionally, consideration must be given to future dilations for growth when determining type of stent and implant diameter (21). While pulmonary artery interventions have a high rate of success in older patients with straightforward lesions, there is still an inherent risk in certain populations accentuating the need for appropriate patient selection prior to intervention (20,22).
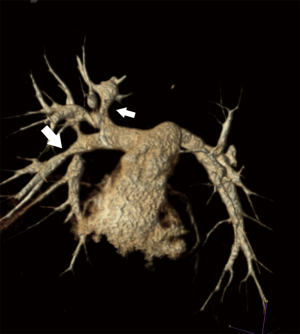
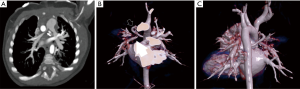
Congenital pulmonary venous abnormalities
The pulmonary veins and left atrium develop separately in early embryogenesis, around 25–27 days post gestation. The pulmonary veins are derived from the splanchnic plexus that connects to both the cardinal veins, which form the brachiocephalic veins and superior vena cava and the umbilicovitelline veins, which form the umbilical vein and portal venous system. By the end of the first month of gestation, a common pulmonary vein has been established which connects to the left atrium. The connections to the splanchnic plexus involute and the common pulmonary vein gradually becomes incorporated into the left atrium so that individual pulmonary veins connect and enter separately (23). Four pulmonary veins are typical however, variability is very common. TTE is the most commonly performed exam for assessment of the pulmonary veins however, limitations based on the acoustic window are common. CTA and MRI therefore are very useful in the evaluation of suspected pulmonary venous anomalies.
Total anomalous pulmonary venous return (TAPVR)
TAPVR results from the failure of fusion of the common pulmonary vein and the left atrium with persistence of connections to the cardinal and umbilicovitelline circulations. This is a cyanotic lesion in which all pulmonary venous return goes to the right heart. Admixture of blood is required, usually through a patent foramen ovale. Four types of TAPVR exist depending on the level of venous drainage. Type I is the classic “snowman” heart on chest radiograph, consist of a supracardiac common “vertical” vein which drains to the left innominate vein. Type II is considered the cardiac type with a common pulmonary vein joining the coronary sinus or right atrium (Figure 7). Type III is the infracardiac variant in which the common pulmonary vein joins the portal vein, the ductus venosus or the inferior vena cava (Figure 8). A mixture of the three types may also occur (24). Type III is associated with asplenia syndrome (bilateral right sidedness). Unobstructed pulmonary venous flow to the right heart, as in type I and II, may result in pulmonary plethora or heart failure. Type III may have obstructed physiology, usually as the vein crosses through the diaphragmatic hiatus leading to pulmonary venous congestion. Obstruction is sometimes related to venous return to the portal vein which then must flow through the liver to return to the heart. Type IV (mixed) is the rarest and consists of veins which drain to two separate locations often above and/or below the diaphragm (25). Prostaglandin therapy is initiated early to help improve systemic and pulmonary perfusion by maintaining a PDA, however; this can lead to worsening pulmonary edema in those patients with obstruction due to increased pulmonary blood flow. Rarely, palliative stent placement has been performed in obstructed vertical veins to relieve pulmonary venous obstruction, especially in very low birth weight infants, temporizing the infant for a more favorable clinical status for definitive repair (26).
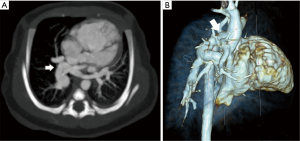
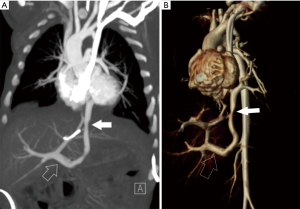
Scimitar syndrome
Scimitar syndrome is a form of partially anomalous pulmonary venous return, also referred to as hypogenetic lung syndrome (Figure 9). The usual triad is a hypoplastic right lung, anomalous right pulmonary venous drainage to the IVC and anomalous systemic arterial supply to the right lower lobe. Hypoplasia or aplasia of the right pulmonary artery may be present. The scimitar refers to the curved anomalous venous drainage resembling the Turkish sword. This venous overload of the right heart can lead to right atrial dilatation and right sided heart failure. This may be seen as acyanotic congestive heart failure in the first months of life as well as more mild cases identified in adulthood with recurrent right lower lobe infections. Treatment in symptomatic patients includes embolization of the systemic arterial supply to the right lung base. This artery typically arises from the celiac trunk, the right phrenic artery or directly from the descending thoracic aorta. Surgical repair is indicated when the pulmonary to systemic blood flow ratio is greater than 1.5 and when the patient has symptoms of pulmonary hypertension (27).
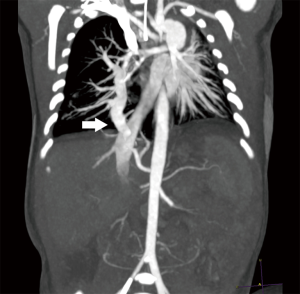
Pulmonary sequestration
Scimitar syndrome should be differentiated from a pulmonary sequestration, which is congenital abnormality of the lungs where lung tissue does not connect to the bronchial tree or pulmonary arteries. Systemic arterial supply from the descending thoracic or abdominal aorta is characteristic. Sequestrations may be extralobar, with its own pleural investment and usual systemic venous drainage (Figure 10); as well as intralobar which does not have its own pleural encasement and has pulmonary venous drainage. The area of lung may look mass like in the absence of aeration and can be prone to repeated infections. Treatment is indicated in symptomatic patients. Extralobar sequestrations typically present in infancy and may be diagnosed antenatally. Intralobar lesions are more typically found in late childhood. Embolization of the aberrant supplying artery has been reported as an alternative to surgical resection in symptomatic patients without infection. Embolization prior to surgical resection to reduce risk of bleeding has also been reported. Typically, occlusion is accomplished with placement of intravascular plugs or coils. Cho et al. reported on a relatively large cohort of patients (n=42) who underwent embolization for sequestration and demonstrated complete regression of the sequestration in only 3 (7%) with partial regression of the lesion in 35 (28).
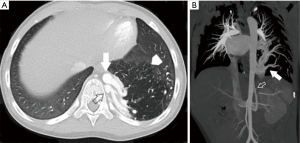
Conclusions
Congenital abnormalities of the pulmonary vascular system are very heterogeneous, with variable presentation, management and outcome based on severity. Many of these anomalies are associated with additional abnormalities of other organ systems or vascular structures. In all cases, multiple imaging modalities are required for complete assessment and treatment planning. Advances in CT and MRI imaging capabilities and techniques have resulted in these imaging modalities becoming an integral part of the diagnostic algorithm. Reduction in radiation both in the interventional laboratory and CT scanner have resulted in much lower risk to patients who often times require multiple studies over the course of their lifetimes. This article provides a brief overview of a range of pulmonary vascular abnormalities with guidelines for imaging and therapy.
Acknowledgements
None.
Footnote
Conflicts of Interest: The authors have no conflicts of interest to declare.
References
- Yanaga Y, Awai K, Nakaura T, et al. Contrast material injection protocol with the dose adjusted to the body surface area for MDCT aortography. AJR Am J Roentgenol 2010;194:903-8. [Crossref] [PubMed]
- Berdon WE, Muensterer OJ, Zong YM, et al. The triad of bridging bronchus malformation associated with left pulmonary artery sling and narrowing of the airway: the legacy of Wells and Landing. Pediatr Radiol 2012;42:215-9. [Crossref] [PubMed]
- Zylak CJ, Eyler WR, Spizsarny DL, et al. Developmental lung anomalies in the adult: radologic-pathologic correlation. RadioGraphics 2002;22:S25-43. [Crossref] [PubMed]
- Xie J, Juan YH, Wang Q, et al. Evaluation of left pulmonary artery sling, associated cardiovascular anomalies, and surgical outcomes using cardiovascular computed tomography angiography. Sci Rep 2017;7:40042. [Crossref] [PubMed]
- Devanagondi R, Peck D, Sagi J, et al. Long-term outcomes of balloon valvuloplasty for isolated pulmonary valve stenosis. Pediatr Cardiol 2017;38:247-54. [Crossref] [PubMed]
- Mullins CE, Pagotta L. Chapter 52: Patent ductus arteriosus. The science and practice of pediatric cardiology. 2nd ed. Williams and Wilkins, 1998:1181-97.
- Udink Ten Cate FE, Sreeram N, Hamza H, et al. Stenting the arterial duct in neonates and infants with congenital heart disease and duct-dependent pulmonary blood flow: a multicenter experience of an evolving therapy over 18 years. Catheter Cardiovasc Interv 2013;82:E233-43. [Crossref] [PubMed]
- Schranz D, Michel-Behnke I, Heyer R, et al. Stent implantation of the arterial duct in newborns with a truly duct-dependent pulmonary circulation: A single center experience with emphasis on aspects of the interventional technique. J Interv Cardiol 2010;23:581-88. [Crossref] [PubMed]
- Hussain A, Al-Aharani S, Muhammed AA, et al. Midterm outcome of stent dilatation of patent ductus arteriosus in ductal-dependent pulmonary circulation. Congenit Heart Dis 2008;3:241-9. [Crossref] [PubMed]
- McMullan DM, Permut LC, Jones TK, et al. Modified Blalock-Taussig shunt versus ductal stenting for palliation of cardiac lesions with inadequate pulmonary blood flow. J Thorac Cardiovasc Surg 2014;147:397-401. [Crossref] [PubMed]
- Mallula K, Vaughn G, El-Said H, et al. Comparison of ductal stenting versus surgical shunts for palliation of patients with pulmonary atresia and intact ventricular septum. Catheter Cardiovasc Interv 2015;85:1196-202. [Crossref] [PubMed]
- Vida VL, Speggiorin S, Maschietto N, et al. Cardiac operations after patent ductus arteriosus stenting in duct-dependent pulmonary circulation. Ann Thorac Surg 2010;90:605-9. [Crossref] [PubMed]
- Leonard H, Derrick G, O’Sullivan J, et al. Natural and unnatural history of pulmonary atresia. Heart 2000;84:499-503. [Crossref] [PubMed]
- Presnell LB, Blankenship A, Cheatham SL, et al. An overview of pulmonary atresia and major aortopulmonary collateral arteries. World J Pediatr Congenit Heart Surg 2015;6:630-9. [Crossref] [PubMed]
- Liu J, Li H, Liu Z, et al. Complete preoperative evaluation of pulmonary atresia with ventricular septal defect with multi-detector computed tomography. PLoS One 2016;11. [Crossref] [PubMed]
- Monge MC, Mainwaring RD, Sheikh AY, et al. Surgical reconstruction of peripheral pulmonary artery stenosis in Williams and Alagille syndromes. J Thorac Cardiovasc Surg 2013;145:476-81. [Crossref] [PubMed]
- Digilio M, Marino B. Clinical manifestations of Noonan syndrome. Images Paediatr Cardiol 2001;3:19-30. [PubMed]
- Collins RT. Cardiovascular disease in Williams syndrome. Circulation 2013;127:2125-34. [Crossref] [PubMed]
- Zussman M, Hirsch R, Beekman RH, et al. Impact of percutaneous interventions for pulmonary artery stenosis in Alagille syndrome. Congenit Heart Dis 2015;10:310-6. [Crossref] [PubMed]
- Lewis MJ, Kennedy KF, Ginns J, et al. Procedural success and adverse events in pulmonary artery stenting. Insights from the NCDR. J Am Coll Cardiol 2016;67:1327-35. [Crossref] [PubMed]
- Cyrstal MA, Ing FF. Pulmonary artery stents: past, present, and future. Prog Pediatr Cardiol 2012;33:151-9. [Crossref]
- Holzer RJ, Gauvreau K, Kreutzer J, et al. Balloon angioplasty and stenting of branch pulmonary arteries: adverse events and procedural characteristics: results of a multi-institutional registry. Circ Cardiovasc Interv 2011;4:287-96. [Crossref] [PubMed]
- Dyer KT, Hlavacek AM, Meinel FG, et al. Imaging in congenital pulmonary vein anomalies: the role of computed tomography. Pediatr Radiol 2014;44:1158-68. [Crossref] [PubMed]
- Donnelly L. Diagnostic Imaging Pediatrics. Amirsys Publishing, 2012.
- Aluja Jaramillo F, Hernandez C, Garzón JP, et al. Infracardiac type total anomalous pulmonary venous return with obstruction and dilatation of portal vein. Radiol Case Rep 2017;12:229-32. [Crossref] [PubMed]
- Kobayashi D, Forbes TJ, Aggarwal S. Palliative stent placement in vertical vein in a 1.4 kg infant with obstructed supracardiac total anomalous pulmonary venous connection. Catheter Cardiovasc Interv 2013;82:574-80. [PubMed]
- Wang CC, Wu ET, Chen SJ, et al. Scimitar syndrome: incidence, treatment and prognosis. Eur J Pediatr 2008;167:155-60. [Crossref] [PubMed]
- Cho MJ, Kim DY, Kim SC, et al. Embolization versus surgical resection of pulmonary sequestration: clinical experiences with a thoracoscopic approach. J Pediatr Surg 2012;47:2228-33. [Crossref] [PubMed]